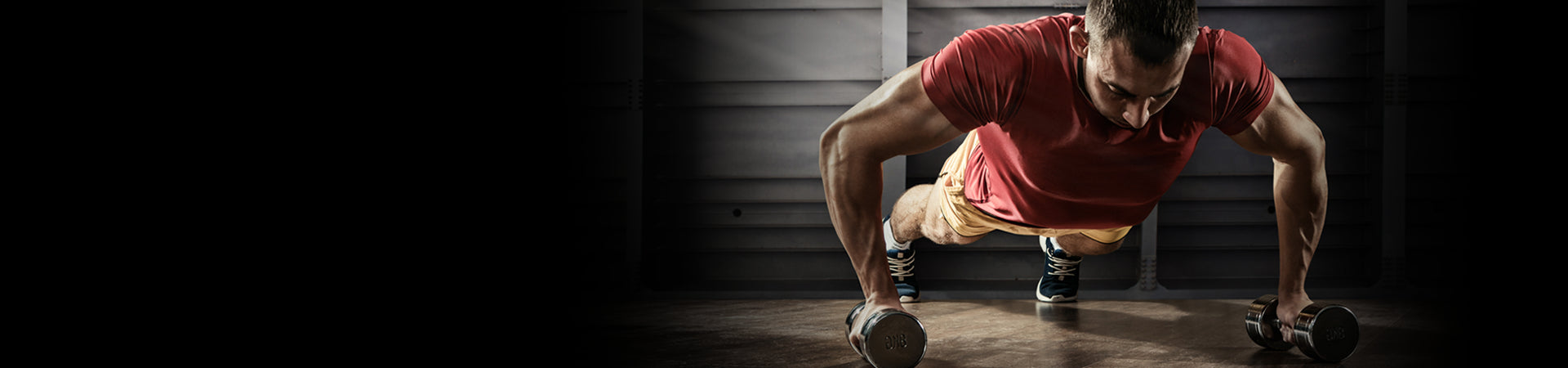
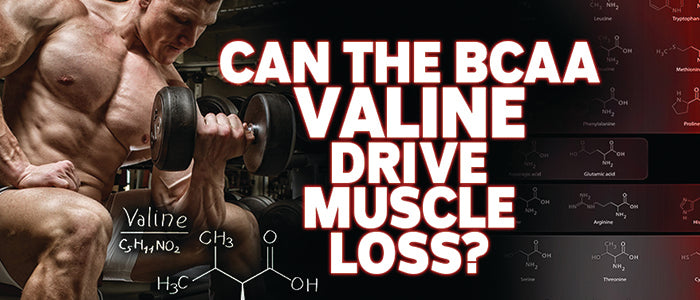
Can the BCAA Valine Drive Muscle Loss?
The branched-chain amino acid (BCAA) is an amino acid with a side chain consisting of covalently linked carbon atoms that form a branch-like structure, thus eliciting their name. There are three BCAAs found in the body: leucine, isoleucine and valine. Supplementation with BCAAs is widespread, mainly because of the apparent ability of BCAAs to increase muscle growth. The trouble is that all three BCAAs do not promote muscle growth equally. In fact, leucine is by far the most potent stimulator of muscle growth, while isoleucine and valine come in a very distant second and third place, respectively.1,2
Leucine Boosts Muscle Growth Without Diminishing Insulin’s Anabolic Action
The major anabolic effect caused by BCAA intake involves insulin. Insulin is one of the most potent muscle-building hormones in the human body, possessing the ability to drastically increase muscle protein synthesis, which enhances muscle growth.3 Insulin achieves this muscle-building effect by binding to the insulin receptor embedded within the muscle cell membrane, setting off a cascade of molecular-signaling events that eventually activates the enzyme mTOR. As a result of mTOR activation, protein synthesis surges within the muscle cell, triggering muscle growth.4 The insulin-signaling cascade is very sensitive to overstimulation, where extraneous activation of the insulin-signaling cascade rapidly triggers negative feedback mechanisms that can result in diminished muscle growth. Several studies have shown that the simultaneous consumption of all three BCAAs can overstimulate the insulin signaling machinery, reducing insulin function and insulin resistance.5,6
Because leucine is the most potent of the BCAAs at boosting insulin activity and muscle growth, leucine is probably the best candidate to overstimulate insulin signaling, making this BCAA the most likely culprit to reduce insulin function. However, leucine consumption alone has actually been shown to rescue insulin-signaling deficiency7, despite the strong influence that leucine has on insulin secretion and signaling activity. Leucine’s strong influence should, in theory, increase the propensity to inhibit insulin function via the previously mentioned negative feedback mechanism that occurs with too much insulin signaling. Although the exact mechanism by which leucine improves insulin function is not completely understood, it appears that leucine’s strong influence on muscle growth generates a greater demand for energy, as muscle tissue is very active metabolically and requires a lot of energy. Because of the increased energy demand, leucine intake also triggers mitochondrial growth within the cell, improving the production of energy by burning more fat.8 The loss of body fat augments the response to insulin signaling, thus overcoming, to a large degree, the negative influence that leucine potentially has on insulin signaling by too much stimulation.
Isoleucine Improves Insulin Function
On the other hand, the remaining two BCAAs, isoleucine and valine, are much less anabolic than leucine— essentially because isoleucine doesn’t stimulate insulin-driven muscle growth at all9 and valine triggers a very weak insulin response.10 So, neither isoleucine or valine should cause insulin resistance either, as neither BCAA likely overstimulates— and thus turns off— insulin function. As for isoleucine, this premise holds true as isoleucine has been shown to actually improve insulin sensitivity by specifically increasing the influx of glucose into the muscle cell and the rate at which glucose is converted into energy within muscle.9 The enhanced capacity to shuttle glucose into the muscle cell reduces the required secretion of insulin from the pancreas. The diminished need for insulin secretion reduces the overall amount of insulin signaling, diminishing feedback inhibition caused by overstimulation, which ultimately augments insulin sensitivity. Incidentally, the enhanced energy production within the muscle cell caused by the isoleucine most certainly enhances muscular performance, likely contributing to muscle growth.
Valine Metabolite Causes Insulin Resistance
Oddly enough, the weak insulinogenic effect caused by valine does not mitigate the negative impact of this BCAA on insulin resistance, as valine has been shown to induce insulin resistance. In fact, higher levels of valine are observed in the blood of diabetic mice, rats and humans11, while mice that were fed a diet without valine improved insulin sensitivity after only one day, while a valine-free diet for an entire week decreased blood glucose levels— indicating improved insulin function.12 So, how could this be? Especially when valine most certainly does not overstimulate, and desensitize, insulin signaling. One potential explanation is that unlike leucine— which avoids insulin resistance by increasing mitochondrial-driven fat loss— valine does not encourage mitochondrial biogenesis, or function, precluding any protective impact associated with fat loss on insulin resistance.
Valine also appears to inhibit insulin signaling in a unique way, likely making this BCAA the most detrimental for muscle growth. This recently discovered effect of valine was reported in the journal Nature Medicine, where research demonstrated that the valine catabolite 3-hydroxyisobutyrate (3-HIB) promoted the accumulation of fat within muscle tissue by directly stimulating fatty acid uptake into the muscle.13 The accumulation of intramuscular fat activates certain signaling cascades within the muscle cell that diminish insulin signaling, leading to insulin resistance. This study further corroborated the impact of the valine metabolite 3-HIB on insulin function by showing that inhibiting the production of 3-HIB in muscle cells prevented the uptake of fat. Interestingly, other studies supporting the negative influence of 3-HIB on insulin signaling have shown that 3-HIB levels are elevated in the muscle of human subjects with diabetes.14,15 Taken together, these results reveal a novel mechanism for valine-induced insulin resistance in muscle tissue that most likely diminishes the capacity to pack on muscle mass.
In closing, the use of BCAAs for muscle growth involves more than merely ingesting a handful of BCAAs after training to get huge. The optimal use of BCAAs requires an informed consumption schedule that prioritizes the use of leucine as this BCAA is, by far, the most potent stimulator of muscle growth— and, perhaps just as importantly, leucine actually enhances the potent anabolic action of insulin. While, on the contrary, isoleucine and valine are much less capable of boosting muscle growth. In addition, it is just as important to consider the potential muscle-depleting impact associated with valine use, as recently discovered evidence demonstrates that valine intake can inhibit insulin signaling, likely reducing your ability to pack on muscle mass.
For most of Michael Rudolph’s career he has been engrossed in the exercise world as either an athlete (he played college football at Hofstra University), personal trainer or as a research scientist (he earned a B.Sc. in Exercise Science at Hofstra University and a Ph.D. in Biochemistry and Molecular Biology from Stony Brook University). After earning his Ph.D., Michael investigated the molecular biology of exercise as a fellow at Harvard Medical School and Columbia University for over eight years. That research contributed seminally to understanding the function of the incredibly important cellular energy sensor AMPK— leading to numerous publications in peer-reviewed journals including the journal Nature. Michael is currently a scientist working at the New York Structural Biology Center doing contract work for the Department of Defense on a project involving national security.
References:
1. Blomstrand E, Eliasson J, et al. Branched-chain amino acids activate key enzymes in protein synthesis after physical exercise. J Nutr 2006;136, 269S-273S.
2. Shimomura Y, Yamamoto Y, et al. Nutraceutical effects of branched-chain amino acids on skeletal muscle. J Nutr 2006;136, 529S-532S.
3. Hillier TA, Fryburg DA, et al. Extreme hyperinsulinemia unmasks insulin's effect to stimulate protein synthesis in the human forearm. Am J Physiol 1998;274, E1067-1074.
4. Guillet C, Prod'homme M, et al. Impaired anabolic response of muscle protein synthesis is associated with S6K1 dysregulation in elderly humans. Faseb J 2004;18, 1586-1587.
5. Newgard CB, An J, et al. A branched-chain amino acid-related metabolic signature that differentiates obese and lean humans and contributes to insulin resistance. Cell Metab 2009;9, 311-326.
6. Tremblay F, Lavigne C, et al. Role of dietary proteins and amino acids in the pathogenesis of insulin resistance. Annu Rev Nutr 2007;27, 293-310.
7. Macotela Y, Emanuelli B, et al. Dietary leucine--an environmental modifier of insulin resistance acting on multiple levels of metabolism. PLoS One 2011;6, e21187.
8. Liang C, Curry BJ, et al. Leucine Modulates Mitochondrial Biogenesis and SIRT1-AMPK Signaling in C2C12 Myotubes. J Nutr Metab 2014;2014, 239750.
9. Doi M, Yamaoka I, et al. Hypoglycemic effect of isoleucine involves increased muscle glucose uptake and whole body glucose oxidation and decreased hepatic gluconeogenesis. Am J Physiol Endocrinol Metab 2007;292, E1683-1693.
10. Fasching P, Ratheiser K, et al. Insulin production following intravenous glucose, arginine, and valine: different pattern in patients with impaired glucose tolerance and non-insulin-dependent diabetes mellitus. Metabolism 1994;43, 385-389.
11. Lynch CJ and Adams SH. Branched-chain amino acids in metabolic signalling and insulin resistance. Nat Rev Endocrinol 2014;10, 723-736.
12. Xiao F, Yu J, et al. Effects of individual branched-chain amino acids deprivation on insulin sensitivity and glucose metabolism in mice. Metabolism 2014;63, 841-850.
13. Jang C, Oh SF, et al. A branched-chain amino acid metabolite drives vascular fatty acid transport and causes insulin resistance. Nat Med 2015;22, 421-426.
14. Avogaro A and Bier DM. Contribution of 3-hydroxyisobutyrate to the measurement of 3-hydroxybutyrate in human plasma: comparison of enzymatic and gas-liquid chromatography-mass spectrometry assays in normal and in diabetic subjects. J Lipid Res 1989;30, 1811-1817.
15. Giesbertz P, Padberg I, et al. Metabolite profiling in plasma and tissues of ob/ob and db/db mice identifies novel markers of obesity and type 2 diabetes. Diabetologia 2015;58, 2133-2143.