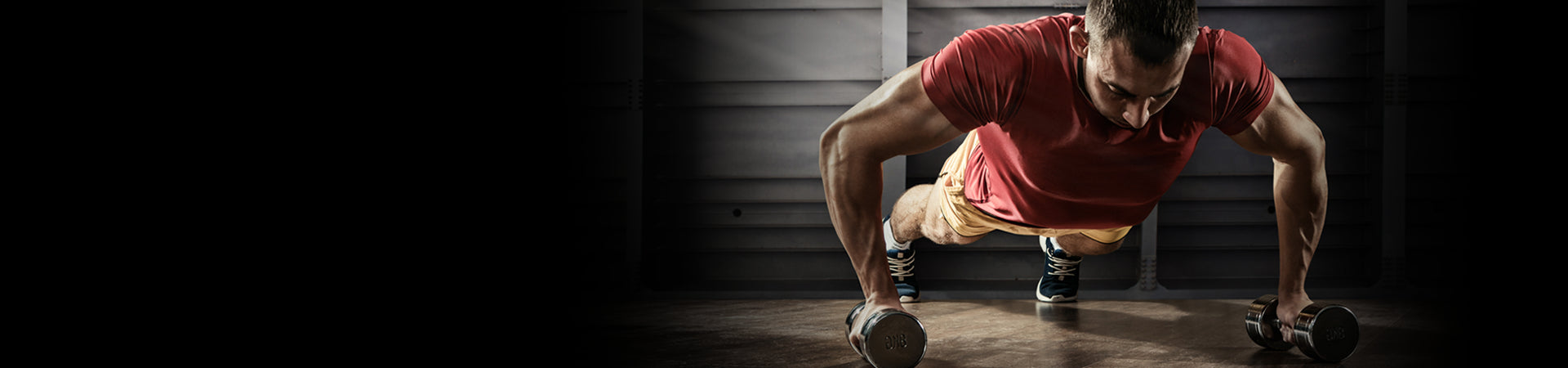
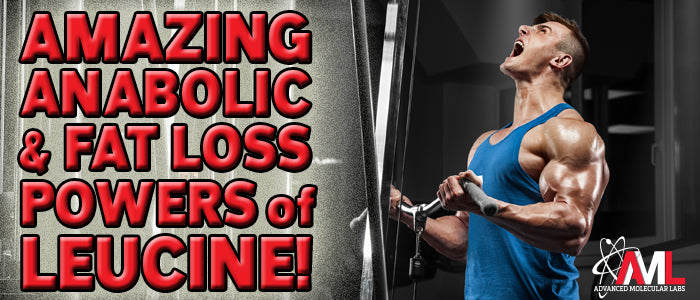
AMAZING ANABOLIC AND FAT-LOSS POWERS OF LEUCINE
By Steve Blechman
Leucine is an essential amino acid that serves as a building block for muscle protein synthesis. Leucine is a powerful anabolic trigger— it’s the most potent branched-chain amino acid (BCAA) and a key activator of the mTOR pathway that is critical for muscle protein synthesis that promotes muscle growth. Leucine has many growth benefits: preventing muscle loss, increasing insulin sensitivity, enhancing fat metabolism and enhancing recovery.
Increases Muscle Protein Synthesis. Muscles increase in size when muscle protein synthesis is greater than muscle breakdown. Protein balance is influenced by muscle tension, physical activity, calories, protein and amino acids. High protein intake temporarily increases protein synthesis in the muscles by activating key biochemical pathways in the cells. When combined with protein, leucine triggers protein synthesis for greater gains.
Leucine, not branched-chain amino acids, is the most important chemical that turns on the mTOR pathway, so it is likely that consuming leucine after exercise would be more effective (and cheaper) than consuming BCAAs. The addition of isoleucine and valine may hinder the benefits of leucine due to competition for transport into muscle cells. The BCAAs share the same active transport system into cells and muscle cells. Indeed, isoleucine and valine have been shown to inhibit absorption of leucine.
Robert R. Wolfe, noted amino acid researcher, said in the Journal of the International Society of Sports Nutrition (2017) that “BCAAs also compete with other amino acids for transport, including phenylalanine, and this competition could affect the intramuscular availability of other EAAs. As a result of competition for transporters, it is possible that leucine alone, for example, could have a transitory stimulatory effect on muscle protein synthesis where the BCAAs fail to elicit such response.”
Studies indicate increases in muscle protein synthesis are dependent on leucine concentration! Leucine stimulates the anabolic effects of muscle protein by itself. A Japanese study published on October 18, 2018 in the journal Nutrients found that taking leucine supplements alone may be better for muscle protein synthesis and more anabolic than leucine from food! Japanese researchers found that blood levels of leucine were higher from pure, free leucine taken alone compared to the same amount of leucine in a meal. Increase in muscle protein synthesis is dependent on leucine concentration. Research has shown that leucine stimulates the anabolic effect of muscle protein on its own (Wilkinson et al., J Physiol, 2013). The Nutrients study showed that, “based on these findings, it is presumed that compared to the intake of protein alone or free amino acids alone, the intake of dietary protein from mixed meals may result in a lower maximum plasma leucine concentration. However, no study to date has investigated the changes in amino acid concentrations after the ingestion of mixed meals in comparison to those after the intake of a similar amount of free amino acids.”
In a randomized crossover study, 10 healthy, young Japanese men underwent tests under different conditions: consuming 2 grams of leucine alone; a mixed meal with 2.15 grams of leucine without any additional leucine supplementation; 2 grams of leucine right after a meal; and the final serving consisted of 2 grams of leucine, 180 minutes after a meal.
The study concluded that “based on the aforementioned discussions, the intake of free leucine alone markedly increased the plasma leucine concentration. However, the increase in leucine concentration after the intake of a mixed meal containing the same amount of leucine was significantly less than that of free leucine intake alone. Moreover, when free leucine was ingested after a mixed meal with the purpose of increasing the plasma leucine concentration, the maximum plasma concentration was attenuated when it was ingested immediately after the mixed meal, despite the fact that the total leucine content was doubled. These results suggest that when free amino acids ingested with the purpose of increasing plasma amino acid concentrations, the timing in relation to the mixed meal intake needs to be considered.”
For best results to use as an anabolic trigger, take 5 grams of leucine (on an empty stomach) 30 minutes before a post-workout meal, or protein shake. A meta-analysis (Nutrition, 2017) that combined the results of seven studies showed that BCAA supplements are best taken after exercise, not before or during exercise (intra-workout.) (Nutrition 2017, 42: 30-36; American Journal of Clinical Nutrition 2016; 104:1594-606; Med Sci Sports Exercise 2011, 43: 2249-2258; Nat Med 2015, 22: 421-426; Biochemical Journal 1996, 100: 7-11; International Journal of Sport Nutrition and Exercise Metabolism, March 2018, 28: 170-177; Amino Acids, June 2008, 35: 147-155; Amino Acids, July 2015, 47: 1389-98).
By taking pure leucine on an empty stomach, you will get a better spike in blood levels than if you take leucine with food, because food can slow leucine’s absorption. The addition of isoleucine and valine may hinder the benefits of leucine due to competition for transport into muscle cells. When leucine is taken on an empty stomach, it’s a powerful metabolic switch that turns on protein synthesis. Leucine increases mTOR activity for several hours after training. When leucine is taken after resistance exercise and before a post-workout, protein-containing meal rich in essential amino acids, it triggers greater protein synthesis for improved recovery and greater gains.
There’s an overwhelming amount of evidence over the years that elevated branched-chain amino acids are associated with obesity and insulin resistance. A most recent meta-analysis study (Acta Diabetologica, November 9, 2018) found that oral BCAA elevates circulating dietary BCAA intake and were positively and inversely related to type 2 diabetes, mellitus and overweight/obesity risk respectively! The researchers said, “Eight articles on randomized clinical trials of oral BCAA supplementation and seven articles on dietary BCAA intake and type 2 diabetes/obesity risks were eligible for inclusion in our meta-analysis.”
A study in the Journal of Physiology (February 2018) suggests that a diet low in BCAAs may help weight loss and prevent the metabolic problems that occur in diabetes and obesity. “We’ve identified an unanticipated role for dietary BCAAs in the regulation of energy balance, and we show that a diet with low levels of BCAAs promotes leanness and good control of blood sugar,” according to an article in Nutraingredients-USA.com, dated December 21, 2017.
“… branched-chain amino acids, or BCAA, had been identified in 2009 as a robust marker of obesity and insulin resistance in humans by Duke researchers led by Christopher Newgard, the director of the Duke Molecular Physiology Institute,” according to ScienceDaily on May 17, 2018 and most recently published, in addition to findings by Duke University researchers in Cell Metabolism also on May 17, 2018. “The association between BCAA and insulin resistance had been present in the literature dating back to a 1969 study that appeared in the New England Journal of Medicine. And they have since been shown to be highly predictive of future diabetes development by the landmark Framingham Heart study.”
“This helps to explain how and why BCAAs are associated with disordered fat metabolism that can lead to type 2 diabetes,” said Newgard, who has worked on BCAA in metabolic disease for more than a decade.
Research has shown that elevated levels of valine are present in the blood of diabetic rats, mice and humans (Nat Rev Endocrinol, 2014). When the mice were fed a diet without valine, insulin sensitivity improved after only one day. Mice on the valine-free diet lasting an entire week showed decreased blood glucose levels, indicating that there was improved insulin function (Metabolism, 2014). It was reported in the journal Nature Medicine in 2015 that valine catabolite 3-hydroxyisobutyrate (3-HIB) promoted the accumulation of fat within muscle tissue by directly stimulating fatty uptake in the muscle. The intramuscular fat activates certain signaling cascades within the muscle cell that diminish insulin signaling, leading to insulin resistance. This study also found that inhibiting the production of 3-HIB prevented the uptake of fat. Other studies support the negative effect of 3-HIB on insulin signaling with elevated 3-HIB in the muscle of human subjects with diabetes (J Lipid Res, 1989; Diabetologia, 2015). An article titled Insulin Resistance, And What May Contribute To It by Lila Abassi and published on the American Council on Science and Health website on March 14, 2016 reported on “… a study published in Nature Medicine, [that] scientists have discovered that 3-hydroxyisobutyrate (3-HIB), one of the intermediate products in the breakdown of the BCAA valine, plays a role in the transport of fatty acids into skeletal muscle cells, which creates fatty muscles — a contributor to insulin resistance.” Abassi also states, “Thus far, it has been a relative mystery as to how BCAAs play a role in insulin resistance. Skeletal muscles display resistance to insulin when there is excess fat inside their cells.” In her closing of the article, Abassi said, “What the researchers found was that 3-HIB acted as a shuttle in muscle cells, allowing blood vessels in skeletal muscle tissue to move fat into skeletal muscle. The more 3-HIB, the more fat was transported — and conversely, when scientists blocked 3-HIB from being made, there was less uptake of fat into skeletal muscle.”
One of the authors of the study, Dr. Zoltan Arany, said to Abassi: “In this study we showed a new mechanism to explain how 3-HIB, by regulating the transport of fatty acids in and out of muscle, links the breakdown of branched-chained amino acids with fatty acid accumulation, showing how increased amino acid flux can cause diabetes.”
More recent studies have confirmed that the branched-chain amino acid valine metabolite 3-HIB is involved in the pathogenesis of insulin resistance in skeletal muscle and might be involved in insulin resistance in humans (Diabetes, July 2017; EbioMedicine, 2018; J Diab Rsch, 2018). Unlike valine, leucine has been shown to improve insulin function. Leucine consumption alone has been shown to rescue insulin-signaling deficiency (PLOS, 2011). A most recent study (Exp Clin Endocrinol Diabetes, 2018) has found that oral administration of leucine improved endothelial function in healthy individuals when infused with glucose. Acute hyperglycemia impairs endothelial function in healthy individuals. This study found that leucine administration prevented hyperglycemia-mediated endothelial function. Unlike leucine, which avoids insulin resistance by increasing mitochondrial-driven fat loss, valine does not encourage mitochondrial biogenesis. Impaired mitochondrial function in skeletal muscle is one of the major predisposing factors to metabolic diseases, such as insulin resistance, type 2 diabetes and cardiovascular disease. Leucine supplementation increases insulin sensitivity by activating SIRT1 activity. SIRT1 is known to “promote mitochondrial biogenesis and oxidative capacity and prevent the mitochondrial dysfunction in skeletal muscle.” (Journal of Nutrition and Metabolism, 2014) Leucine may also attenuate adiposity and promote weight loss during energy restriction (Nutrition 2006, Diabetes, 2007). These effects are in part by activating the SIRT1-dependent pathway, stimulating mitochondrial biogenesis and increased oxygen consumption (Nutrition Metabolism, 2008). Mitochondrial biogenesis and SIRT1 expression in skeletal muscle has also been shown to increase lifespan in middle-aged mice (Cell Metabolism, 2010). As far as isoleucine is concerned, unlike valine, it has been shown to improve insulin sensitivity by increasing glucose into muscle cells (Am J Physiol Endocrinol Metab, 2007).
In conclusion, the research shows that leucine is a powerful anabolic trigger and enhances protein synthesis and can promote muscle growth and recovery. Also, leucine supplementation can improve mitochondrial biogenesis and function, increase insulin sensitivity and may also enhance fat loss and improve lean body mass.
References:
- Yoshii at al. Nutrients 2018, 10(10), 1543; https://doi.org/10.3390/nu10101543Effect of Mixed Meal and Leucine Intake on Plasma Amino Acid Concentrations in Young Men/
Rahimi MH, Shab-Bidar S et al. Branched-chain amino acid supplementation and exercise-induced muscle damage in exercise recovery: A meta-analysis of randomized clinical trials. Nutrition 2017.
Chad M Kerksick, Colin D Wilborn, Michael D. ISSN exercise & sports nutrition review update: research & recommendations.
Roberts, et al. Journal of the International Society of Sports Nutrition 2018 15:38 https://doi.org/10.1186/s12970-018-0242-y 1 June 2018
Robert R. Wolfe. Branched-chain amino acids and muscle protein synthesis in humans: myth or reality? Journal of the International Society of Sports Nutrition201714:30 https://doi.org/10.1186/s12970-017-0184-9
Szmelcman S, Guggenheim K. Interference between leucine, isoleucine and valine during intestinal absorption. Biochemical Journal 1966;100(1):7-11.
Wilkinson, DJ et al. Effects of leucine and its metabolite beta-hydroxy-beta-methylbutyrateonhumanskeletalmuscleproteinmetabolism.J.Physiol.2013,591,2911–2923.
Cummings NE, Williams EM, Kasza I, Konon EN et al. (2018), Restoration of metabolic health by decreased consumption of branched‐chain amino acids. J Physiol, 596: 623-645. doi:10.1113/JP275075
Duke University. "Diabetes researchers find switch for fatty liver disease: Carbs, fats and protein: One molecule to rule them all?" ScienceDaily, 17 May 2018. www.sciencedaily.com/releases/2018/05/180517113847.htm
PJ White, RW McGarrah, PA Grimsrud, S Tso, W Yang, JM Haldeman, C Newgard et al. The BCKDH Kinase and Phosphatase Integrate BCAA and Lipid Metabolism via Regulation of ATP-Citrate Lyase. Cell Metabolism, 2018; DOI: 10.1016/j.cmet.2018.04.015
NutraIngredients-USA.com, December 17, 2017. Nathan Gray. Could dropping specific amino acids from diet be key to weight loss?
CB Newgard, J An, JR Bain et al. A Branched-Chain Amino Acid-Related Metabolic Signature that Differentiates Obese and Lean Humans and Contributes to Insulin Resistance Cell Metab. 2009 April; 9(4): 311-326. doi:10.1016/j.cmet.2009.02.002
Zheng Y, Li Y, Qi Q et al. Cumulative consumption of branched-chain amino acids and incidence of type 2 diabetes. Int J Epidemiol. 2016; 45: 1482-92.
Isanejad M, LaCroix AZ, Thomson CA et al. Branched-chain amino acid, meat intake and risk of type 2 diabetes in the Women’s Health Initiative. Br J Nutr. 2017; 117:1523-30.
Newgard CB, An J, Bain JR et al. A branched-chain amino acid-related metabolic signature that differentiates obese and lean humans and contributes to insulin resistance. Cell Metab. 2009; 9: 311-26
Tremblay F, Krebs M, Dombrowski L et al. Overactivation of S6 kinase 1 as a cause of human insulin resistance during increased amino acid availability. Diabetes. 2005; 54: 2674-84.
Tremblay F, Krebs M, Dombrowski L et al. Overactivation of S6 kinase 1 as a cause of human insulin resistance during increased amino acid availability. Diabetes. 2005; 54: 2674-84.
Lee CC, Watkins SM, Lorenzo C et al. Branched-chain amino acids and insulin metabolism: The Insulin Resistance Atherosclerosis Study (IRAS). Diabetes Care.2016; 39: 582-8.
Yoon M-S. The emerging role of branched-chain amino acids in insulin resistance and metabolism. Forum Nutr 2016; 8: 405-17.
Abdul-Ghani MA, Tripathy D and DeFronzo RA. Contributions of beta-cell dysfunction and insulin resistance to the pathogenesis of impaired glucose tolerance and impaired fasting glucose. Diabetes Care. 2006; 29:1130-9.
Jang C, Oh, SF, et al A branched-chain amino acid metabolite drives vascular fatty acid transport and causes insulin resistance. Nat. Med. 22, 421-426
Sara B Seidelmann, Brian Claggett, Susan Cheng, Mir Henglin, Amil Shah, Lyn M Steffen, Aaron R Folsom, Eric B Rimm, Walter C Willett, Scott D Solomon, Dietary carbohydrate intake and mortality: a prospective cohort study and meta-analysis, The Lancet Public Health, 2018, ISSN 2468-2667, https://doi.org/10.1016/S2468-2667(18)30135-X.
Zhaoping Li, Professor of Medicine, UCLA, US National Library of Medicine www. ClinicalTrials.gov Identifier: NCT02684565 Last Update Posted: February 12, 2018 https://clinicaltrials.gov/ct2/show/NCT02684565
Lotta LA, Scott RA, Sharp SJ, Burgess S, Luan J, et al. (2016) Genetic Predisposition to an Impaired Metabolism of the Branched-Chain Amino Acids and Risk of Type 2 Diabetes: A Mendelian Randomisation Analysis. PLOS Medicine 13(11): e1002179. https://doi.org/10.1371/journal.pmed.1002179
Avogaro A and Bier DM (1989) Contribution of 3-hydroxyisobutyrate to the measurement of 3-hydroxybutyrate in human plasma: comparison of enzymatic and gas-liquid chromatography-mass spectrometry assays in normal and in diabetic subjects. J Lipid Res 30, 1811-1817
Giesbertz P, Padberg I et al. (2015) Metabolite profiling in plasma and tissues of ob/ob and db/db mice identifies novel markers of obesity and type 2 diabetes. Diabetologia 58, 2133-2143.
“Insulin Resistance, And What May Contribute to It,” by Lila Abassi on March 14, 2016. American Council on Science & Health https://www.acsh.org/news/2016/03/14/branched-chain-amino-metabolite-a-culprit-in-insulin-resistance
Lydia-Ann LS Harris, Gordon I Smith, Bruce W Patterson, Raja S Ramaswamy et al. Alterations in 3-Hydroxyisobutyrate and FGF21 Metabolism Are Associated With Protein Ingestion–Induced Insulin Resistance Diabetes 2017;66:1871-1878 https://doi.org/10.2337/db16-1475
Mardinoglu, Adil et al. Elevated Plasma Levels of 3-Hydroxyisobutyric Acid Are Associated With Incident Type 2 Diabetes EBioMedicine, Volume 27, 151-155, Jan. 2018.
Ulrika Andersson-Hall, Carolina Gustavsson, Anders Pedersen, Daniel Malmodin, Louise Joelsson, and Agneta Holmäng, “Higher Concentrations of BCAAs and 3-HIB Are Associated with Insulin Resistance in the Transition from Gestational Diabetes to Type 2 Diabetes,” Journal of Diabetes Research, vol. 2018, Article ID 4207067, 12 pages, 2018. https://doi.org/10.1155/2018/4207067.
Macotela Y, Emanuelli B, Bang AM et al. (2011) Dietary leucine – an environmental modifier of insulin resistance acting on multiple levels of metabolism. PLoS One 6, e21187.
Cunxi Nie, Ting He, Wenju Zhang, Guolong Zhang, Xi Ma. Branched Chain Amino Acids: Beyond Nutrition Metabolism. Int J Mol Sci. 2018 Apr; 19(4): 954. Published online 2018 Mar 23. doi: 10.3390/ijms19040954
Y Zhang, K Guo, RE LeBlanc, D Loh, GJ Schwartz and Y Yu. Increasing Dietary Leucine Intake Reduces Diet-Induced Obesity and Improves Glucose and Cholesterol Metabolism in Mice via Multimechanisms. Diabetes Jun 2007, 56 (6) 1647-1654; DOI: 10.2337/db07-0123
Deirdre K Tobias, Clary Clish, Samia Mora, Jun Li, Liming Liang, Frank B. Hu, JoAnn E. Manson, Cuilin Zhang. Dietary Intakes and Circulating Concentrations of Branched-Chain Amino Acids in Relation to Incident Type 2 Diabetes Risk Among High-Risk Women with a History of Gestational Diabetes Mellitus Clinical Chemistry Aug 2018, 64 (8) 1203-1210; DOI: 10.1373/clinchem.2017.285841
McCormack S.E, Shaham O, McCarthy MA, Deik AA, Wang TJ, Gerszten RE, Clish CB, Mootha VK, Grinspoon SK and Fleischman A. (2013), Branched‐chain amino acids and IR in children. Pediatric Obesity, 8: 52-61. doi:10.1111/j.2047-6310.2012.00087.x.
Sina S Ullrich, Penelope CE Fitzgerald, Gudrun Schober, Robert E Steinert, Michael Horowitz, Christine Feinle-Bisset; Intragastric administration of leucine or isoleucine lowers the blood glucose response to a mixed-nutrient drink by different mechanisms in healthy, lean volunteers, The American Journal of Clinical Nutrition, Volume 104, Issue 5, 1 November 2016, Pages 1274-1284, https://doi.org/10.3945/ajcn.116.140640.
Haufe S. et al. Branched-chain and aromatic amino acids, insulin resistance and liver specific ectopic fat storage in overweight to obese subjects. Nutrition, Metabolism and Cardiovascular Diseases , Volume 26, Issue 7, 637-642
Li H, Xu M, Lee J, He C and Xie Z. (2012). Leucine supplementation increases SIRT1 expression and prevents mitochondrial dysfunction and metabolic disorders in high-fat diet-induced obese mice. American Journal of Physiology - Endocrinology and Metabolism, 303(10), E1234-E1244. http://doi.org/10.1152/ajpendo.00198.2012
Chunzi Liang, Benjamin J Curry, Patricia L Brown and Michael B. Zemel, “Leucine Modulates Mitochondrial Biogenesis and SIRT1-AMPK Signaling in C2C12 Myotubes,” Journal of Nutrition and Metabolism, vol. 2014, Article ID 239750, 11 pages, 2014. https://doi.org/10.1155/2014/239750.
Binder E, Bermúdez-Silva FJ, André C, Elie M, Romero-Zerbo SY, et al. Leucine Supplementation Protects from Insulin Resistance by Regulating Adiposity Levels (2013). Leucine Supplementation Protects from Insulin Resistance by Regulating Adiposity Levels. PLOS ONE 8(9): e74705. https://doi.org/10.1371/journal.pone.0074705
Banerjee, Jheelam et al. Activation of the AMPK/Sirt1 pathway by a leucine-metformin combination increases insulin sensitivity in skeletal muscle, and stimulates glucose and lipid metabolism and increases life span in Caenorhabditis elegans. Metabolism - Clinical and Experimental, Volume 65, Issue 11, 1679-1691
Jiao J, Han S-F, Zhang W, Xu J-Y, Tong X, Yin X-B, Qin L-Q (2016). Chronic leucine supplementation improves lipid metabolism in C57BL/6J mice fed with a high-fat/cholesterol diet. Food & Nutrition Research, 60, 10.3402/fnr.v60.31304. http://doi.org/10.3402/fnr.v60.31304
Cholsoon Jang, Sungwhan F Oh, Shogo Wada, Glenn C Rowe, Laura Liu, Mun Chun Chan, James Rhee, Atsushi Hoshino, Boa Kim, Ayon Ibrahim, Luisa G Baca, Esl Kim, Chandra C Ghosh, Samir M Parikh, Aihua Jiang, Qingwei Chu, Daniel E Forman, Stewart H Lecker, Saikumari Krishnaiah, Joshua D Rabinowitz, Aalim M Weljie, Joseph A Baur, Dennis L Kasper & Zoltan Arany. Published: 07 March 2016. A branched-chain amino acid metabolite drives vascular fatty acid transport and causes insulin resistance. Nature Medicine volume 22, pages 421-426 (2016)
D’Antona G, Ragni M, Cardile A, Tedesco L, Dossena M, Bruttini F et al. (2010). Branched-chain amino acid supplementation promotes survival and supports cardiac and skeletal muscle mitochondrial biogenesis in middle-aged mice. Cell Metab. 12, 362-372. doi: 10.1016/j.cmet.2010.08.016
Szmelcman S, Guggenheim K. Interference between leucine, isoleucine and valine during intestinal absorption. Biochemical Journal. 1966;100(1):7-11.
Robert R. Wolfe. Branched-chain amino acids and muscle protein synthesis in humans: myth or reality? Journal of the International Society of Sports Nutrition201714:30 https://doi.org/10.1186/s12970-017-0184-9
L Deldicque, C Sanchez Canedo and S Horman. Antagonistic effects of leucine and glutamine on the mTOR pathway in myogenic C2C12 cells. Amino Acids, 2008, Volume 35, Number 1, Page 147
Amy Hector, Stuart M. Phillips. Protein Recommendations for Weight Loss in Elite Athletes: A Focus on Body Composition and Performance. Int J Sport Nutr Exerc Metab. 2017
Rahimi MH, Shab-Bidar S, Mollahosseini M, Djafarian K. Nutrition 2017. Leucine, Not Total Protein, Content of a Supplement Is the Primary Determinant of Muscle Protein Anabolic Responses in Healthy Older Women, The Journal of Nutrition, nxy091, June 13, 2018
Branched-chain amino acid supplementation and exercise-induced muscle damage in exercise recovery: A meta-analysis of randomized clinical trials.
Jiao J, Han S.F, Zhang W, Xu JY, Tong X, Yin XB, Yuan LX, Qin LQ (2016). Chronic leucine supplementation improves lipid metabolism in C57BL/6J mice fed with a high-fat/cholesterol diet. Food & nutrition research, 60, 31304. doi:10.3402/fnr.v60.31304
Macotela Y, Emanuelli B, Ba˚ng AM, Espinoza DO, Boucher J, Beebe K, et al. Dietary leucine an environmental modifier of insulin resistance acting on multiple levels of metabolism. PLoS One 2011; 6: e21187.
Zhang Y, Guo K, LeBlanc RE, Loh D, Schwartz GJ, Yu YH. Increasing dietary leucine intake reduces diet-induced obesity and improves glucose and cholesterol metabolism in mice via multimechanisms. Diabetes 2007; 56: 164754.
Khan M, Joseph F. Adipose tissue and adipokines: the association with and application of adipokines in obesity. Scientifica (Cairo) 2014; 2014: 328592
Zemel MB, Bruckbauer A. Effects of a leucine and pyridoxine containing nutraceutical on fat oxidation, and oxidative and inflammatory stress in overweight and obese subjects. Nutrients 2012; 4: 52941
Ricoult SJ, Manning BD. The multifaceted role of mTORC1 in the control of lipid metabolism. EMBO Rep 2013; 14: 24251
Freudenberg A, Petzke KJ, Klaus S. Comparison of high protein diets and leucine supplementation in the prevention of metabolic syndrome and related disorders in mice. J Nutr Biochem 2012; 3: 152430.
Vaughan RA, Garcia-Smith R, Gannon NP, Bisoffi M, Trujillo KA, Conn CA. Leucine treatment enhances oxidative capacity through complete carbohydrate oxidation and increased mitochondrial density in skeletal muscle cells. Amino Acids 2013; 45: 90111.
Zhang Y et al. (2007) Increasing dietary leucine intake reduces diet-induced obesity and improves glucose and cholesterol metabolism in mice via multimechanisms. Diabetes 56(6):1647-1654
Okekunle AP, Zhang M, Wang Z. et al. Dietary branched-chain amino acids intake exhibited a different relationship with type 2 diabetes and obesity risk: a meta-analysis. Acta Diabetol (2018). https://doi.org/10.1007/s00592-018-1243-7
Binder E, Bermúdez-Silva FJ, André C, Elie M, Romero-Zerbo SY, et al. Leucine Supplementation Protects from Insulin Resistance by Regulating Adiposity Levels (2013) Leucine Supplementation Protects from Insulin Resistance by Regulating Adiposity Levels. PLOS ONE 8(9): e74705. https://doi.org/10.1371/journal.pone.0074705
Binder E, Bermúdez‐Silva FJ, Elie M, Leste‐Lasserre T, Belluomo I, Clark S, Duchampt A, Mithieux G and Cota D. (2014) Leucine supplementation modulates fuel substrates utilization and glucose metabolism in previously obese mice. Obesity, 22: 713-720. doi:10.1002/oby.20578
Li H, Xu M, Lee J, He C and Xie Z (2012). Leucine supplementation increases SIRT1 expression and prevents mitochondrial dysfunction and metabolic disorders in high-fat diet-induced obese mice. American journal of physiology. Endocrinology and metabolism, 303(10), E1234-44.
Pedroso JA, Zampieri TT and and Donato J. (2015). Reviewing the Effects of L-Leucine Supplementation in the Regulation of Food Intake, Energy Balance, and Glucose Homeostasis. Nutrients, 7 (5), 3914-37. https://dx.doi.org/10.3390%2Fnu7053914
Caoileann H Murphy, Nelson I Saddler, Michaela C Devries, Chris McGlory, Steven K Baker and Stuart M Phillips. Leucine supplementation enhances integrative myofibrillar protein synthesis in free-living older men consuming lower- and higher-protein diets: a parallel-group crossover study, The American Journal of Clinical Nutrition, Volume 104, Issue 6, 1 December 2016, Pages 1594-1606, https://doi.org/10.3945/ajcn.116.136424
Yadao DR, MacKenzie S and Bergdahl A. (2018) Reducing branched‐chain amino acid intake to reverse metabolic complications in obesity and type 2 diabetes. J Physiol, 596: 3455-3456. doi:10.1113/JP276274